We’ve come to rely on ultrasound technology to provide non-invasive, real-time insights into our bodies. But have you ever wondered how it works? Let’s explore the physics and instrumentation behind it. Ultrasound waves are mechanical waves that propagate through media, affected by acoustic impedance, and attenuate as they travel through tissues. Transducers convert electrical energy into sound waves, which are sent into the body and return as echoes, processed into electrical signals. As we explore further, we’ll uncover the intricacies of image reconstruction, advanced modalities, and the exciting future of ultrasound technology.
Key Takeaways
- Ultrasound waves are mechanical waves that propagate through media, transmitting energy via particle vibration, causing compressions and rarefactions.
- The pulse-echo principle involves sending ultrasound pulses into the body, and echoes return to the transducer, converted into electrical signals.
- Ultrasound transducers convert electrical energy into sound waves through the piezoelectric effect, generating high-frequency sound waves for diagnostic imaging.
- Image quality optimization involves signal attenuation compensation, filtering, and artifact correction to enhance image clarity and provide accurate diagnoses.
- Frequency range of ultrasound is 2-18 MHz, with higher frequencies yielding better resolution but lower penetration, affecting diagnostic capabilities.
Fundamentals of Ultrasound Waves
We’ll start by exploring the core characteristics of ultrasound waves, which are a type of mechanical wave that propagates through a medium, like air, water, or human tissue.
These waves transmit energy through the vibration of particles, causing a series of compressions and rarefactions. When ultrasound waves encounter different materials, their propagation is affected by the acoustic impedance of each medium.
This property, which depends on the density and elasticity of the material, determines how much energy is reflected, absorbed, or transmitted. Understanding how ultrasound waves interact with different tissues is essential for medical imaging applications.
High-Frequency Sound Wave Generation
As we explore the world of ultrasound technology, generating high-frequency sound waves is an essential step in producing diagnostic images.
To achieve this, we rely on a vital component: the ultrasound transducer. This device converts electrical energy into high-frequency sound waves, which is made possible by the piezoelectric effect.
When an electric current is applied to the transducer, it causes the piezoelectric material to deform, generating sound waves at frequencies beyond human hearing.
These high-frequency sound waves are then transmitted into the body, where they interact with tissues and reflect back to the transducer, allowing us to capture detailed images of internal structures.
Transducer Design and Construction
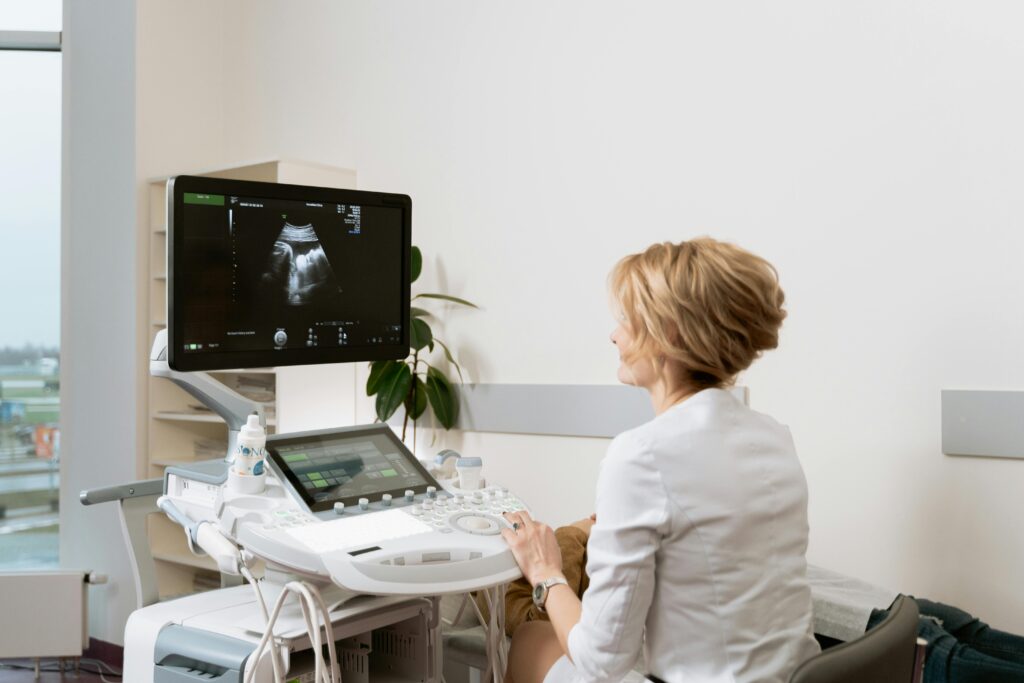
The ultrasound transducer’s design and construction play an essential role in guaranteeing efficient high-frequency sound wave generation and accurate image production.
We need a transducer that can efficiently convert electrical energy into sound waves and vice versa. To achieve this, we use a piezoelectric crystal, which changes shape when an electric current is applied. This change in shape creates the high-frequency sound wave.
The crystal is typically mounted on a backing material and connected to an ultrasound probe, which is the component that comes into contact with the patient’s body. The probe’s shape and size are designed to optimize image quality and patient comfort.
Pulse-Echo Principle and Signal Processing
Once we’ve generated high-frequency sound waves using our transducer, it’s time to detect the echoes that bounce back from the patient’s body and process them into a usable image.
This is where the pulse-echo principle comes in. We send a pulse of ultrasound into the body, and then wait for the echoes to return to the transducer. The transducer converts these echoes into electrical signals, which are then processed to create an image.
We use signal processing techniques to amplify, filter, and analyze these signals, removing noise and artifacts to produce a clear image of the patient’s internal structures.
Frequency and Wavelength in Ultrasound
We explore the fundamental properties of ultrasound, starting with frequency and wavelength. These two properties are essential in understanding how ultrasound works.
- The frequency of ultrasound typically ranges from 2-18 MHz, with higher frequencies providing better resolution but lower penetration depths.
- Ultrasound frequencies above 10 MHz are usually used for superficial structures, while lower frequencies are used for deeper tissues.
- The wavelength of ultrasound is inversely proportional to its frequency, meaning higher frequencies have shorter wavelengths.
- A shorter wavelength allows for better resolution, but it also increases the likelihood of attenuation.
Understanding the relationship between frequency and wavelength is critical in optimizing ultrasound imaging for specific applications.
Attenuation and Absorption of Ultrasound Waves
Ultrasound waves don’t travel indefinitely; they’re gradually weakened as they interact with tissues. This weakening occurs due to two primary mechanisms: attenuation and absorption.
Attenuation is the loss of ultrasound wave intensity as it travels through a medium, and it’s largely influenced by the medium’s impedance. When ultrasound waves encounter tissues with different impedance values, some of the energy is reflected, scattered, or absorbed, leading to attenuation.
Absorption, on the other hand, is the conversion of ultrasound energy into heat, which is then dissipated by the tissue. As a result, the ultrasound wave’s intensity decreases, making it essential to take into account these factors when using ultrasound technology for diagnostic or therapeutic purposes.
Reflection and Refraction of Ultrasound Waves
As we probe deeper into the behavior of ultrasound waves, we find that their interaction with tissues doesn’t just lead to attenuation and absorption.
When an ultrasound wave encounters a boundary between two tissues with different propagation speeds, it can be reflected or refracted.
- Reflection occurs when the wave hits a surface at a perpendicular angle, bouncing back into the original tissue.
- Refraction occurs when the wave passes from one tissue to another, changing direction due to differences in propagation speed.
- The angle of incidence equals the angle of reflection.
- The amount of reflection and refraction depends on the acoustic impedance mismatch between the two tissues.
Understanding reflection and refraction is essential for accurate image formation in ultrasound imaging.
Doppler Effect and Blood Flow Analysis
When an ultrasound wave encounters moving blood cells, its frequency changes, a phenomenon known as the Doppler effect. This change in frequency is directly proportional to the velocity of the moving cells.
We can harness this principle to analyze blood flow in vessels. In Doppler mode, the ultrasound machine detects the frequency shift and converts it into a velocity measurement. This information is then used to create a color Doppler image, where blood flow is depicted in shades of red and blue, indicating direction and velocity.
Instrumentation Components and Their Functions
We’re now going to explore the heart of an ultrasound machine, examining the various instrumentation components that work together to produce high-quality images.
These components are essential in understanding ultrasound physics and instrumentation, as they play an important role in capturing and processing ultrasound data.
- Transducer: Converts electrical energy into sound waves and vice versa, acting as both a transmitter and receiver.
- Pulse generator: Produces high-voltage electrical pulses to drive the transducer.
- Scan converter: Interprets and processes the received ultrasound signals, generating a 2D image.
- Image processor: Enhances and refines the image, applying filters and corrections as needed.
- Display: Presents the final ultrasound image to the operator, allowing for real-time assessment and diagnosis.
Ultrasound Beam Formation and Steering
Forming and steering an ultrasound beam is a complex process that relies on the careful manipulation of sound waves.
We achieve ultrasound beam formation by arranging multiple transducer elements in a specific pattern. Each element converts electrical energy into ultrasound waves, which then pass through the tissue.
By carefully timing the emission of these waves, we can control the direction of the resulting beam. To target a specific area, we adjust the delay between wave emissions, allowing the ultrasound waves to pass perpendicular to the target.
This precise control enables us to focus the beam on a specific region, creating a high-resolution image.
Image Reconstruction and Display
As the ultrasound beam interacts with the body, it generates a vast amount of data in the form of echoes and reflections. This data is then processed and reconstructed into a visual representation, allowing us to interpret the internal structures of the body.
- The reconstructed data is displayed on the ultrasound screen in real-time, providing instant feedback to the operator.
- The most common mode of display is B-mode, which represents the amplitude of the echoes as brightness on a two-dimensional grid.
- The resulting image is a 2D representation of the 3D anatomy, allowing us to visualize the internal structures in real-time ultrasound.
- The image reconstruction process involves correcting for artifacts, compensating for signal attenuation, and applying filters to enhance image quality.
- The final image is then optimized for diagnostic interpretation, enabling healthcare professionals to make accurate diagnoses and treatment decisions.
Advanced Ultrasound Modalities and Applications
Beyond traditional B-mode imaging, ultrasound technology has evolved to encompass a range of advanced modalities and applications that greatly enhance diagnostic capabilities.
We’re now able to visualize complex anatomy and physiology in unprecedented detail. Advanced ultrasound modalities like 3D ultrasound allow for precise volumetric measurements and enhanced visualization of complex structures.
High-frequency ultrasound, on the other hand, enables us to image superficial tissues and small parts with remarkable resolution.
These advanced modalities have opened up new avenues for diagnosis and treatment in various fields, including cardiology, oncology, and musculoskeletal medicine.
As we continue to push the boundaries of ultrasound technology, we’re excited to see the impact it will have on patient care and outcomes.
Frequently Asked Questions
What is ultrasound and how does it work?
Ultrasound is a diagnostic imaging technique that uses high-frequency sound waves to create images of organs and structures inside the body. It operates on the principles of ultrasound physics, where a transducer emits ultrasound waves into the body. These waves travel through various tissues and are reflected back to the transducer as echoes. The ultrasound machine then processes these echoes to produce a visual representation, known as an ultrasound image.
What are the basic principles of ultrasound physics?
The fundamental principles of ultrasound physics include the generation and propagation of ultrasound waves, reflection, refraction, and the doppler effect. Sound waves travel through different media at different speeds, which is influenced by the acoustic impedance of the tissues. When these sound waves hit a boundary between different tissues, some of the waves are reflected back, which allows for imaging. The doppler effect is utilized to measure the velocity of blood flow by analyzing the frequency shift of the reflected waves.
What is a transducer in the context of ultrasound?
A transducer is a crucial component of the ultrasound machine. It converts electrical energy into ultrasound waves using the piezoelectric effect. The transducer also receives the echoes reflected from the tissues, converting them back into electrical signals for image processing. Different types of transducers are designed for various applications, including linear, curved, and phased array types, each suited for specific imaging tasks.
What is ultrasound and how does it work?
Ultrasound is a medical imaging technique that uses high-frequency sound waves to create images of organs and structures inside the body. The physics of ultrasound revolves around the transmission, reflection, and interpretation of ultrasound waves. The ultrasound machine emits sound waves through a transducer, which then listens for the echoes that bounce back from tissues. These echoes are processed to produce real-time images that allow healthcare professionals to diagnose and monitor various medical conditions.
What is a transducer and what role does it play in ultrasound?
A transducer is a vital component of an ultrasound system that converts electrical energy into ultrasound waves and vice versa. Using the piezoelectric effect, the transducer emits sound waves into the body and receives the echoes that return, allowing for the formation of an ultrasound image. Different types of transducers are used depending on the specific ultrasound application, such as imaging shallow structures or deeper organs.
What is the significance of MHz in ultrasound?
The term mhz refers to megahertz, a unit that measures frequency. In ultrasound, the frequency of the sound waves plays a crucial role in determining the quality of the ultrasound image. Higher frequencies (typically 5-15 mhz) provide better resolution but have less penetration, making them ideal for imaging superficial structures. Conversely, lower frequencies (1-5 mhz) penetrate deeper but produce lower-resolution images, which is beneficial for viewing deeper organs.
Conclusion
As we conclude this whirlwind tour of ultrasound physics and instrumentation, we’ve unraveled the mysteries of high-frequency sound waves, transducer design, and pulse-echo principles. We’ve navigated the complex landscape of frequency, wavelength, and beam formation. And, like a master chef combining ingredients, we’ve mixed and matched these components to create stunning images and advanced modalities. Now, with this foundation in place, the possibilities are endless – and the future of ultrasound is bright!